An indicator of the presence of water-soluble organic matter in an environmental sample can be measured chemically as chemical oxygen demand (COD). In the same sample, there is some organic matter that is degraded aerobically by measuring biochemical oxygen demand [1], also called biological oxygen demand (BOD) [2]. Thus, the BOD concentration is less than, and the maximum is equal to COD. The BOD/COD ratio indicates the level of biodegradability of organic matter in wastewater, which has a COD concentration of 400 mg/L to 2500 mg/L [3], with a detention time of 30 days for optimized power generation [4]. This ratio is also used as a measure of the degree of available energy and toxicity to microorganisms in activated sludge [5], and applied to compostable solid waste [6] and for monitoring purposes [7]. Previously, there were three zones on the BOD/COD ratio [8], viz. toxic, biodegradable and stable levels, based on the characteristic effect of the ratio.
The toxic zone limits the concentration of organic matter that is harmful to microbes. A BOD/COD ratio level below 0.1 characterizes this zone. The minimum concentrations of BOD and COD need to be determined through an acute toxicity test on the processing microbes [9] and for the biological process [10].
The biodegradable zone limits the concentration of organic matter that microbes can degrade. This zone has a BOD/COD ratio limit above the toxic zone to a maximum of 1.0. The maximum concentrations of BOD and COD are practically below the toxic concentration but need to be determined through biodegradability tests [11] as applied to trimeprazine degradation [12], such as respiration tests for bioremediation [13] and activated sludge test protocol [14].
The stable zone limits the concentration of organic matter that can be safely discharged into the environment without a significant impact on the overall quality of the environment. BOD and COD concentrations are below the biodegradable zone with a BOD/COD ratio below 0.1. The ratio of BOD/COD for the stable and toxic zones is the same, approaching 0.1. It is necessary to include the concentration of each parameter to distinguish which is stable and which is toxic. The stable zone had BOD and COD concentrations below 100 mg/L, while the toxic zone exceeded 500 mg/L [8]. For example, if BOD is 10 mg/L and COD 100 mg/L, a BOD/COD ratio of 0.1 is stable. For BOD 500 mg/L, COD 5,000 mg/L, then a BOD/COD ratio of 0.1 is toxic.
The difficulty in implementing detoxification or stabilization is mainly in the fluctuating concentrations of BOD and COD in the toxic and stable zones. This paper presents in-depth insights into the changing characteristics of the zone through the BOD/COD ratio to solve the problem. It is complemented by a novel approach for sequential change from the toxic zone to the biodegradable zone, called detoxification. Further, it reaches a stable zone, where the concentration of organic matter decreases, called stabilization. Within the framework of these two mechanisms, this study aims to formulate methods for improving indicators of organic matter, BOD and COD, attempting to enhance detoxification and stabilization of organic matter. It can be useful in applying wastewater treatment and remediation of polluted environments. One can also use this formulation to determine the system's feasibility and configuration of wastewater treatment and remediation of polluted environments.
This literature research defines two processes of detoxification and stabilization of organic matter. Both are the basis for searching for literature sources and selection criteria. The selected formula is related to these two processes. The organization of this study is visualized in Figure 1, which describes the materials and methods to achieve the objectives. The flow of this assessment starts from the detoxification process to stabilization.
Framework of the materials and methods of this study
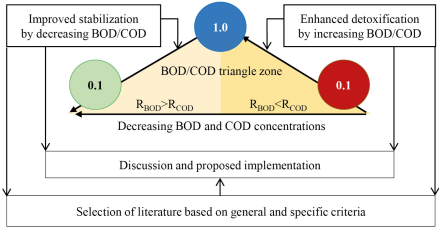
The literature research is divided into three topics. The first is to provide facts about increasing the detoxification of organic matter by increasing the BOD/COD ratio from less than 0.1 to 1.0, where BOD removal (RBOD) is less than COD removal (RCOD). The second topic is a continuation of the achievement of the first topic to improve the stability of organic matter by reducing the BOD/COD ratio to the lowest possible level, where RBOD is more than RCOD. In all processes of changing the BOD/COD ratio, the concentrations of BOD and COD must decrease. The third topic is a discussion on the results of the two topics, along with the proposed implementation.
The literature on all topics was selected using the Mendeley Reference Manager tool with the search keywords: BOD, COD, detoxification, biodegradation, stabilization, organic matter. Furthermore, the results were screened using the following general criteria: open access journals, at least abstract, in English, and the longest time of publication, 20 years. Each collected piece of literature was reviewed regarding abstracts, methods, results, and conclusions. In particular, the authors deliberately set out the following criteria to select the literature differentiated between enhanced detoxification or biodegradation and improved stability.
Selected literature on enhanced detoxification met the criteria: untreated wastewater or polluted environment, main content of organic compounds, one of the BOD and COD has a concentration of at least 1.0 g/L, the BOD/COD ratio close to 0.1, and processing results show an increase in BOD/COD.
In the improved stability range using the criteria: treated wastewater, organic matter content, BOD or COD concentration below 1.0 g/L, maximum BOD/COD ratio 1.0, the results showed a decrease in the BOD/COD ratio.
The supporting literature on the topic of the proposed implementation meets the criteria: advances in biological treatment, including microbial wastewater treatment, bioremediation and phytoremediation in polluted environments.
Of the hundreds of papers published in various journal media, and using the selection criteria above, 110 selected articles were obtained, the oldest one published in 2006.
Each detoxification, biodegradation, and stabilization of organic materials requires a formula for calculating changes in the concentration of organic materials in terms of substance and effect on biota. The formula applied includes the removal of BOD and COD, detoxification, and stabilization.
It is well known that the decrease in the concentration of a substance is expressed as the removal efficiency in eq. (1), that has been used for the treatment of palm oil refinery effluent [15], removal of BOD and COD from tannery wastewater [16], and removal efficiency of lignin [17], as well as treatment of industrial wastewater with gamma irradiation [18]:
(1)
where Re is the efficiency of reducing the concentration of the substance, U is the concentration of the untreated substance, and T is the concentration of the treated substance. Eq. (1) calculates the removal efficiency of BOD and COD concentrations.
In expressing detoxification, the concentration of a substance, resulting in the elimination of half the response units of biota, is expressed as effect concentration fifty (EC-50), which is applied to assess the toxicity of ochratoxin [19], calculation of effective concentrations [20], and responses of the earthworm [21]. When the effect of a substance produces a biota response in the form of death or elimination, it is known as lethal concentration fifty (LC-50). A substance with a low EC-50 is more toxic than another substance with a high EC-50 for assessing toxicological interactions [22], the effects of acute ammonia exposure [23], and acute and chronic aqueous toxicity of vanadium [24]. These units are needed to compare the quality of untreated and treated wastewater, as is the substance removal unit in eq. (1).
In a well-run wastewater treatment process, the treated wastewater is less toxic than the untreated wastewater, which means the EC-50 untreated wastewater is lower than the treated wastewater. When eq. (1) is used to calculate the detoxification efficiency, the result is negative. Therefore, a toxicity unit (TU) was introduced, which is the reciprocal of the EC-50 in the same treatment unit. Accordingly, the TU is expressed as eq. (2):
(2)
Eq. (2) was derived from research works concerning the toxic effects of three active pharmaceutical ingredients [25], toxicity decline of diclofenac [26], ecological assessment of coal mine and metal mine drainage [27], and toxicity assessment method [28]. It has been used for municipal wastewater treatment plants [29] by classifying the level of acute toxicity unit (TUa) as follows:
TUa < 0.4 | : | No acute toxicity |
0.4 < TUa < 1 | : | Slight acute toxicity |
1 ≤ TUa < 10 | : | Acute toxicity |
10 ≤ TUa < 100 | : | High acute toxicity |
TUa ≥ 100 | : | Very high acute toxicity |
The classification shows that efforts to increase the detoxification of a substance must result in a decrease in TU from an untreated substance to a treated substance. Accordingly, the detoxification of substances is expressed in eq. (3) as follows [30]:
(3)
where D is the detoxification efficiency of reducing the concentration of the substance, TUu is the toxicity unit of the untreated substance, and TUt is the toxicity unit of the treated substance, which results in a positive efficiency value.
In wastewater and the environment, there are many substances and/or intentionally added new substances to stimulate biological processes. In this condition, it is necessary to assess the interaction effect of many substances, which can be additive, synergistic, antagonistic, and independent. An empirical study [25] formulates the mixture effect in eq. (4) as follows:
(4)
where TUi equals the sum of each substance's toxicity units. The mixed effect can be evaluated according to the values of M and Mo in eq. (5) under the following conditions:
(5)
The mixed effect assessment is stated as follows:
M < 1 | : | Synergistic |
M = 1 | : | Simple additive |
M = Mo | : | Independent |
M > Mo | : | Antagonism |
Mo > M > 1 | : | Partial additive |
The applications of stabilization of organic matter in question include a decrease in the concentration of carbon sources in compost processing [31], respiration and enzymatic activities [32], and furfural biodegradation with microbial consortium [33]. The concentration decreases to levels which indicate that microbes do not get a carbon source (BOD) for their respiration process. A respiration inhibition test can be carried out using the OCSPP 850.3300 guidelines [14] to measure microbial respiration towards exhaustion. The test results show the volume of carbon dioxide produced by the respiration of wastewater processing biota. The results can be further calculated regarding respiratory efficiency, similar to the eq. (1) and presented in eq. (6) as follows:
(6)
where R is the efficiency of respiration, Ru is the respiration result of the untreated substance, and Rt is the respiration result of the treated substance.
The results of previous studies can be classified into processing to increase the detoxification of organic substances and their stability. Each process is carried out physically, chemically and biologically, involving microbes and plants.
Physical methods to increase biodegradability can be carried out using the following treatment. The toxicity of diclofenac organic substances in wastewater was reduced by ultraviolet A (UVA) radiation at a wavelength of about 300-320 nm. The product of the photo transformation (e.g., quinones and dimers [34] and diclofenac [35]) is adsorbed by the hydrophobic absorbent material polyvinylidene difluoride (PVDF). The combination of UVA radiation and PVDF adsorption can eliminate many other pollutants in wastewater at the same time [26].
Chemically, the simple method is adding a biodegradable carbon source [36], such as glucose [37], into the wastewater treatment unit. The addition of this biodegradable and water-soluble substance instantly increases the BOD concentration and the BOD/COD ratio.
The addition of multiple chemicals has been conducted by researchers [25] using a mixture of triclosan and ibuprofen exposed to the test organism Vibrio fischeri. Triclosan and ibuprofen produced TUi of 1.426 and 2.633, respectively. With eq. (4) obtained the M value of 4.059, and then using eq. (5) obtained a Mo value of 1.541 (= 4.059/2.633); thus, the mixture is antagonistic. The same organism has been studied, which has different effects due to single exposure to petroleum oil [38] and leachate containing a mixture of organic matter and heavy metals [28]. In addition, exposing other organisms to the combined effect of acute and chronic toxicity [39] and heavy metal mixtures [40] produces different toxicity units due to different organisms and toxicants.
A study investigated the electrochemical oxidation method for leachate containing BOD and COD of more than 1 g/L, each with a BOD/COD ratio of 0.04. The electrode used was carbon graphite with sodium sulphate electrolyte. Under optimal operating conditions, the BOD removal efficiency was slightly lower than the COD removal efficiency, so the method did not significantly increase the BOD/COD ratio [41]. This method is important for further similar studies to confirm the same problem adequately. However, electrochemical oxidation using aluminium-aluminium, aluminium-iron and iron-iron electrodes increased the BOD/COD ratio at COD concentrations above 1 g/L [42].
Wastewater from the pulp and paper industry was treated by electrocoagulation using an aluminium anode cell and a water cathode so that aluminium ions acted as an in-situ coagulant. The concentrations of BOD and COD in wastewater were 530 mg/L and 1.357 g/L, respectively, and after undergoing electrocoagulation, BOD decreased by 53% and COD by 71% [17]. The fact shows that the electrocoagulation of aluminium increases the BOD/COD ratio from 0.39 to 0.63, in this case, detoxifying the wastewater.
Experiments on pretreatment using ozone (O3) were carried out to oxidize organic matter-rich wastewater from a biomethane refinery. The characteristics of untreated wastewater had a BOD/COD ratio of less than 0.2. With the application of ozone oxidation, the BOD/COD ratio increased to 0.6. In addition, the treated wastewater positively affected seed germination by up to 35% [43]. With the same process, wastewater from the textile production process, which before ozone oxidation had a BOD/COD ratio of about 0.2, can be increased to about 0.3 [44]. Another experiment related to pulp and paper mill wastewater containing aliphatic and lignin-derived compounds. The application of the oxidation process using ozone increased the BOD/COD ratio by 0.2 [45].
Olive oil wastewater containing high organic matter with a BOD concentration of about 20 g/L and a COD concentration close to 70 g/L was treated by the Fenton oxidation process. Under optimal operating conditions, BOD and COD concentrations decreased by 60% and 80%, respectively [46]. Likewise, wastewater from the natural gas processing industry had BOD and COD concentrations of about 8 g/L and 50 g/L, respectively. The BOD/COD ratio was less than 0.2 but increased to about 0.4 after the Fenton oxidation process [47]. Similar results in increasing the BOD/COD ratio were obtained by the Fenton oxidation process applied to industrial wastewater from producing metronidazole antibiotics [48]. In addition, the electro-Fenton process can increase the biodegradability of leachate with high organic matter content by increasing the BOD/COD ratio from 0.3 to 0.5 [49]. This method was confirmed in a similar experiment using photo-Fenton oxidation [50].
One can apply a biological method to increase detoxification by adding biostimulants in organic [51] and inorganic substances [52]. The type of stimulant is different between stimulants for microbes and plants. Microbial stimulants include Rhizobium and Trichoderma. Plant stimulants include protein hydrolysates, humate substances, seaweed extracts, and biopolymers, such as chitosan [53]. Stimulants are needed by biota to stimulate metabolic processes and increase nutrient absorption and resistance to abiotic environmental stresses. These properties can promote the growth of biota and, therefore, can improve the performance of the detoxification of organic matter.
Besides biostimulation, detoxification can be improved by employing bioaugmentation [54] and in combination [55], viz. addition of a bacterial seed mixture into a microbial treatment unit or a polluted environment unit. The potential of fungi was tested for bioaugmentation of activated sludge treatment against olive mill wastewaters. The BOD and COD contents of activated sludge were 30 mg/L and 100 mg/L, respectively. The fungi Phanerochaete chrysosporium and Trametes versicolor were added to the processing to degrade lignin while releasing various enzymes. Bioaugmentation treatment with P. chrysosporium or T. versicolor resulted in BOD and COD content of about 20 mg/L and 40 mg/L, respectively, with an increase of 0.3 to 0.5 of the BOD/COD ratio [56].
These results were strengthened by the study of dairy wastewater treatment, which had the same BOD and COD contents, however, using the bioaugmentation of the fungi Aspergillus niger, Mucor hiemalis and Galactomyces geotrichum. Before bioaugmentation processing, the BOD/COD ratio was 0.09-0.22; after bioaugmentation, the BOD/COD ratio was 0.4-0.65 [57]. The experiments on wastewater treatment containing aromatic and aliphatic organic matter were carried out using a mixture of Micrococcus, Pseudomonas, Bacillus and Nocardia microbes. The removal of BOD and COD concentrations was 70%-90% without bioaugmentation but more than 90% after bioaugmentation [58]; this means that bioaugmentation increased the BOD/COD ratio. Bioaugmentation was also applied to fermented wastewater containing high organic matter of cocoa bean [59], cheese [60], and composting [61] to speed up the process.
In addition to microbial processes, biological methods involve phytotechnological processes. This treatment uses plants to reduce the concentration of pollutants without negatively affecting the plants themselves. Wastewater from the emulsion paint industry was treated using Azolla pinnata, Eichhornia crassipes and Lemna minor plants. The content of untreated wastewater showed BOD and COD concentrations of 2.4 g/L and 8.4 g/L, respectively, and a BOD/COD ratio of 0.28. After passing the wastewater and plant detention time for six weeks, the BOD/COD ratio increased by 0.32 and 0.30 by A. pinnata and L. minor, respectively. Except for E. crassipes, the BOD/COD ratio was the same as the BOD/COD ratio of untreated wastewater, even though the plant could reduce the concentration of BOD and COD by 55% each [62].
Meanwhile, the treatment of water polluted with wastewater using Lemna minor showed a 95% COD reduction greater than the 68% decrease in BOD [63]. This plant shows its ability to detoxify organic wastewater. Likewise, a helophytic grass, Phragmites australis, can reduce phenol pollution of water. It can lower the BOD concentration from 423 mg/L to 223 mg/L and COD from 1.057 g/L to 476 mg/L [64], thereby increasing the BOD/COD ratio from 0.4 to 0.47.
One can apply physico-chemical methods to increase stabilization. Urban wastewater containing BOD and COD of 173 mg/L and 266 mg/L, respectively, was treated through a precipitation process in a sedimentation tank. The results of the optimal process operating conditions showed concentrations of 26 mg/L and 50 mg/L, respectively [65]. The physical treatment proved that the sedimentation tank could stabilize organic wastewater containing BOD and COD below 300 mg/L, which decreased the BOD/COD ratio by 0.13.
School wastewater treatment was carried out physically using screening, sand filters and ultraviolet (UV) irradiation. BOD and COD concentrations were below 100 mg/L with a BOD/COD ratio of 0.45. The BOD/COD ratio decreased after screening by 0.44, sand filtration by 0.39, and UV irradiation by 0.27 [66]. These results are sufficient for implementing on-site sanitation systems for various domestic wastewater sources.
Wastewater from crude palm oil refining was treated using a tannin polymer coagulant. Untreated wastewater contained BOD and COD concentrations of 518 mg/L and 1.35 g/L, respectively. With the addition of tannin, the concentrations of BOD and COD were 12 mg/L and 150 mg/L, respectively [15]. Tannin coagulant application decreased 0.3 of BOD/COD, indicating a very efficient stabilization of organic matter.
Wastewater from the pulp and paper industry was treated using ferric chloride as a coagulant. The initial contents of BOD and COD were 530 mg/L and 1.357 g/L, respectively. The decrease in BOD and COD after chemical coagulation was 42% and 32%, respectively [17]. The reduction of the BOD/COD ratio from 0.39 to 0.33 by ferric chloride coagulation indicated that ferric chloride plays a role in stabilizing the organic matter of wastewater.
The tannery wastewater, containing BOD of 92 mg/L and COD of 576 mg/L, was treated using an adsorbent ZnO nanopowder (ZnO-NP) derived from the synthesis of Spinacia oleracea leaf extract and Zn(NO3)2 at 500 oC. Under optimal process operating conditions, both the adsorbent dose and pH and with a contact time of 20 minutes produced BOD and COD of 17 mg/L and 500 mg/L, respectively. The decrease in the values of both parameters continued for up to 100 minutes, which resulted in BOD and COD concentrations of 8 mg/L and 288 mg/L, respectively [16]. The chemical adsorption treatment reduced the ratio of BOD/COD from 0.16 to at least 0.03, which indicated the occurrence of stabilization of organic matter. However, it was less effective in reducing COD.
Another approach to stabilizing organic matter is to use biological processes. For example, Livestock wastewater contained organic matter with BOD and COD concentrations of about 600 mg/L and 1.8 g/L, respectively. The treatment used a combination of physico-chemical and biological processes, including the activated sludge method, filtration and ozonation. The combination process results showed that the concentrations of BOD and COD were 12 mg/L and 128 mg/L, respectively. This process stabilised organic matter, indicated by lowering BOD/COD from 0.3 to 0.09 [67].
Conventional microbial processes using the extended aeration sludge method can reduce the concentration of BOD and COD by 85% and 81%, respectively [65]. The decrease in BOD concentration was greater than that of COD, which indicated a decrease in the BOD/COD ratio as a sign of stabilization of organic matter quality. Likewise, the treatment of leachate with a BOD/COD ratio of around 0.50.76 using a two-stage membrane bioreactor can reduce the BOD/COD ratio to 0.11 [68].
In another example, palm oil industrial wastewater has BOD and COD characteristics of about 400 mg/L and 800 mg/L, respectively. The treatment of wastewater using Vetiver plants with a detention time of 14 days resulted in BOD of around 15 mg/L and COD of around 550 mg/L [69]. The treatment process using plants for wastewater showed a decrease in BOD/COD towards 0.03, meaning that it stabilized organic matter.
The treatment of textile-industry wastewater was studied using the aquatic plants Eichhornia crassipes (E), Pistia stratiotes (P), and Spirodela polyrhiza (S), as well as the algae Nostoc (N). The characteristics of untreated wastewater had BOD and COD concentrations of 358 mg/L and 721 mg/L, respectively, with a BOD/COD ratio of 0.49. Plant processing was carried out separately for each plant and mixture (M). For plant mixtures, the weight of N was half the weight of each macrophyte. After processing for seven days, the BOD/COD ratio by E, P, S, N and M were 0.37, 0.42, 0.44, 0.52, and 0.59, respectively [70]. Thus, wastewater treatment by macrophytes reduced the BOD/COD ratio at less than 1 g/L of each concentration. Similar results were obtained in many previous studies, i.e., wetland treating piggery wastewater [71], phytoremediation potential for dairy wastewater [72], phytoremediation of dyeing activities [73], reduction of organic matter [74], organic matter stabilization in integrated wastewater treatment plant [75], macrophytes for biogas generation [76], treatment of domestic wastewater [77], and treatment of industrial mines wastewater [78].
However, microphytes increase the BOD/COD ratio. Even with a mixture of all four biotas, the result of the process increases the BOD/COD ratio over untreated wastewater. In this case, the algae Nostoc determines the change in the status of the BOD/COD ratio. These results are an important concern for further confirmation so that microphytes can be utilized to increase the biodegradability and detoxification of organic matter contained in wastewater.
This discussion analyses the important results of physical, chemical, and biological methods found in previous studies. Further important is the analysis of new sequential methods of detoxification and stabilization, which can be implemented for wastewater treatment and/or remediation of polluted environments.
Table 1 summarises the current methods of increasing and decreasing the BOD/COD ratio based on the results of previous empirical studies. Their application by separate treatment depends on the initial concentration of BOD and COD. If a single treatment system is still being developed, it is important to consider the following two options. Multi-chemical additions do not have to be a mixture of organic materials; a mixture of inorganic materials may work well to get closer to reality. Some types of microphytes can play a dual role - detoxification and stabilization, thus promising the completion of processes in one treatment system.
Summary of empirical research results on detoxification and stabilization of organic matter
Methods | Detoxification | Stabilization |
---|---|---|
Physico-chemical | UV radiation |
Conventional sedimentation, sand filtration |
Biological | Biostimulants |
Combined conventional processes |
The categorisation of BOD and COD concentrations at the 1.0 g/L limit must be re-evaluated. There are findings that the BOD/COD ratio increases if each concentration is below 1 g/L as applied for bioaugmentation processes of dairy wastewater [56], activated sludge [57], industrial wastewater [58], and urban wastewater [79]. In assessing the changes in the characteristics of organic matter from toxic to biodegradable and stable, important is the change in the BOD/COD ratio regardless of the concentration of each parameter. Detoxification or biodegradability is indicated by increasing the BOD/COD ratio, while the opposite indicates stabilization.
The results of previous empirical studies showed that TU is the specificity of the interaction between toxicants and organisms. Consequently, the comparison of TU is not between one treatment unit and another but between untreated and treated toxicants in the same treatment unit. In terms of negative effects, what is meant by the antagonistic effect of the mixture is a decrease in negative effects, meaning an increase in detoxification.
The difference in increasing the ratio of BOD/COD depends on the overall quality of the wastewater, and the operating conditions, so one cannot compare it between one treatment and another. It is important to pay attention to the increase in the BOD/COD ratio as a sign of increased detoxification or biodegradation of organic matter. Likewise, the decrease in the BOD/COD ratio is significant due to the stabilization process, indicating a decrease in respiration. Therefore, the comparison between the different treatments is through the detoxification efficiency and respiration efficiency.
With two results of the acute toxicity test and respiration test, one can decide which method to choose, of course, on the greatest detoxification efficiency and respiration efficiency. In this position, one has obtained the most technically feasible configuration of the biological treatment system. The system configuration may be only biological treatment with extended detention time, such as microbubble technology [80], constructed wetlands [81], activated sludge [82], detention basin [83], anaerobic co-digestion [84], and organic waste composting [85]. One can apply series units, such as an anaerobic baffled reactor used to treat fishmeal wastewater [86], domestic wastewater [87], swine wastewater [88], and various wastewater sources [89]. It can also be a series of different treatments, for example, chemical treatment [90] for detoxification followed by phytotechnological treatment, such as series-type flow constructed wetland [91] for stabilization.
In contrast to the current status, a new approach is proposed regarding a sequential process, detoxification followed by stabilization under minimum BOD and COD concentrations above 500 mg/L. Once a system configuration is decided, one needs to perform a start-up procedure for optimising process operations, which becomes the operational monitoring framework, as presented in Figure 2. The detoxification and stabilization required in wastewater treatment and/or remediation of polluted environments are enhanced by emphasizing the BOD and COD parameters in wastewater quality.
Framework for sequential detoxification and stabilization processes
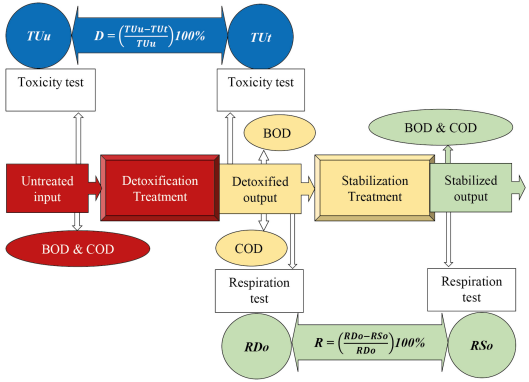
Figure 2 shows an untreated wastewater input sample subjected to laboratory tests on BOD and COD parameters. The same sample is also tested for acute toxicity using the test biota in the detoxification treatment, yielding an untreated toxicity unit (TUu). After passing the holding time specified in the detoxification treatment, it is the output of the detoxified wastewater, which has biodegradable organic matter. The treated wastewater is tested for BOD and COD parameters in this position. An acute toxicity test employing the test biota in detoxification treatment is also performed, resulting in the treated toxicity unit (TUt). The following criteria indicate the success of increasing detoxification:
TUt < TUu by using eq. (2), and confirmed to be antagonistic using eq. (4) and eq. (5); after that, detoxification efficiency (D) is calculated using eq. (3) regardless of the result;
BOD removal efficiency < COD removal efficiency by using eq. (1); as a result, output BOD/COD ratio > input BOD/COD ratio.
As shown in Figure 2, the detoxification output becomes the input of the stabilization treatment with known concentrations of BOD and COD. The detoxified sample must undergo a respiration test with the biota used in stabilization treatment, resulting in the respiration of detoxified output (RDo). The detoxified output samples undergo an acute toxicity test using the biota used in the detoxification treatment and a respiration test using the biota used in the stabilization treatment, thus reaffirming the result.
After passing the detention time specified in the stabilization treatment, the output of the stabilized wastewater is stable. In this last position, in addition to the BOD and COD parameter tests, the stabilized sample must undergo a respiration test with the biota used in the stabilisation treatment, resulting in the respiration stabilized output (RSo). The following criteria indicate the success of increasing stabilization:
RSo < RDo; respiration efficiency (R) is calculated using eq. (6) regardless of the result;
BOD removal efficiency ? COD removal efficiency using eq. (1); the resulting relationship is output BOD/COD ratio ? input BOD/COD ratio.
In practice, the framework of Figure 2 is not only carried out at the start-up of wastewater treatment and environmental remediation. It is also important and necessary in monitoring the rapid toxicological assessment of oil spills [92], evaluation of toxicity reduction [93], monitoring of the ecotoxicological hazard potential [94], and biological wastewater treatment [95] (in addition to the operation of the microbial treatment unit). Acute toxicity test for monitoring allows obtaining information on changes in the quality of BOD and COD faster than by parametric measurements of the two parameters. Changes that may occur can be a clue for improving the operating process in the shortest possible time early warning sensor [96], microbial sensors [97], microbial fuel cells [98], and treatment process using a trend analysis [99]. However, toxicity tests do not replace parametric tests as details of wastewater quality; therefore, parametric tests are still required to be measured periodically.
In addition to the implementation in wastewater treatment, the described approach can be useful in bioremediation and phytoremediation for polluted environments [100] comparing laboratory and field remediation [101], using mixed plant operations [102], and remediation of toxic organic matter [103]. Applications have also been reported for hilly landscaping [104] and landscape aquatic plants [105].
Bioremediation is a microbial recovery process separate from phytoremediation. The former can be carried out on soil and water without plants if implemented by indigenous microorganisms [106] and microbial consortium [107]. However, the latter is the recovery process using plants. It is always related to bioremediation in the plant root zone through rhizosphere processes [108], rhizodegradation [109], and soil conditioners for rhizodegradation improvement [110].
One can highlight the following important findings based on empirical research studies regarding water-soluble organic matter indicators such as BOD and COD quality parameters.
Above all, the comparison of detoxification is not appropriate for different wastewater treatment systems but is suitable for comparing untreated and treated wastewater in the same system.
An increase in the BOD/COD ratio is a criterion for increasing the detoxification of an environmental sample and indicating its biodegradability. The maximum concentration of each parameter does not inhibit processing biota. Therefore, acute toxicity testing is needed as a tool for detoxification processes. Detoxification efficiency is calculated from untreated and treated wastewater's acute toxicity test results.
At concentrations of BOD and COD in the level of biodegradation and a decrease in the ratio of BOD/COD, the organic matter is stabilised to a concentration level with no effect on biota and safe disposal into the environment. Therefore, respiration inhibition testing is needed as a tool for the stabilization process. Respiration efficiency is calculated from the results of the respiration inhibition test on untreated and treated wastewater.
There are two groups of methods to enhance detoxification and stabilization: physico-chemical and biological. The selected method uses the greatest efficiency of detoxification based on acute toxicity tests, plus the greatest efficiency of respiration based on respiration inhibition tests. The key result is a sequential wastewater treatment system configuration, which is technically feasible for the minimum concentration of BOD and COD above 500 mg/L. With the same treatment, these tests are applicable to solve the problems of the polluted water and soil environment so that the configuration of the remediation system is technically correct.
Eventually, this study also provides recommendations for further empirical research. First, the electrochemical oxidation method requires samples containing BOD and COD of less than 1 g/L to prove whether or not organic matter can be detoxified because previous studies have shown that detoxification did not work at higher concentrations. Second, it is essential to identify the determining biota in the detoxification process. Third, further work is needed on biostimulation and bioaugmentation for the ecosystem related to the biota community.
- https://www.usgs.gov/special-topics/water-science-school/science/biological-oxygen-demand-bod-and-water?qt-science_center_objects=0#qt-science_center_objects , 2018,
- https://www.sciencedirect.com/topics/agricultural-and-biological-sciences/biochemical-oxygen-demand , 2016,
Determination of optimum retention time in an aircathode single-chamber microbial fuel cell batch-mode reactor by comparing different substrate types and concentrations ,Process Safety and Environmental Protection , Vol. 162 ,pp 694705 , 2022, https://doi.org/https://doi.org/10.1016/j.psep.2022.04.023
, Enhancement of Power Generation and Organic Removal in Double Anode Chamber Designed Dual-Chamber Microbial Fuel Cell (DAC-DCMFC) ,Water , Vol. 13 (21), 2021, https://doi.org/https://doi.org/10.3390/w13212941
, Biodegradability of wastewater determines microbial assembly mechanisms in full-scale wastewater treatment plants ,Water Research , Vol. 169 ,pp 115276 , 2020, https://doi.org/https://doi.org/10.1016/j.watres.2019.115276
, Determination of the specific energy of mixed waste decomposition in compost solid phase microbial fuel cells (CSMFCS) ,International Journal of Civil Engineering and Technology , Vol. 9 (11),pp 13161324 , 2018
, Estimating the Biodegradability of Treated Sewage Samples Using Synchronous Fluorescence Spectra ,Sensors (Basel) , Vol. 11 (8),pp 73827394 , 2011, https://doi.org/https://doi.org/10.3390/s110807382
, Review on BOD, COD and BOD/COD ratio: A Triangle Zone for Toxic, Biodegradable and Stable Levels ,International Journal of Academic Research , Vol. 2 (4),pp 235239 , 2010
, Ecotoxicological Effect of Aged Wood Leachates to Aquatic Organisms ,Water , Vol. 12 (8), 2020, https://doi.org/https://doi.org/10.3390/w12082091
, Towards Energy Efficient Onsite Wastewater Treatment ,Civil Engineering Journal , Vol. 6 (7), 2020, https://doi.org/https://doi.org/10.28991/cej-2020-03091542
, Influence of short- and long-term exposure on the biodegradation capacity of activated sludge microbial communities in ready biodegradability tests ,Environ. Sci.: Water Res. Technol. , Vol. 7 (1),pp 107121 , 2021, https://doi.org/https://doi.org/10.1039/D0EW00776E
, Trimeprazine is enantioselectively degraded by an activated sludge in ready biodegradability test conditions ,Water Res , Vol. 141 ,pp 5764 , 2018, https://doi.org/https://doi.org/10.1016/j.watres.2018.05.008
, Laboratory tests for aerobic bioremediation of the contaminated sites in the Czech Republic ,Plant, Soil and Environment , Vol. 66 (20205),pp 191199 , 2020, https://doi.org/https://doi.org/10.17221/673/2019-PSE
, , OCSPP 850.3300: Modified Activated Sludge, Respiration Inhibition Test EPA 712-C-014 , 2012
, Treatment of Palm Oil Refinery Effluent Using Tannin as a Polymeric Coagulant: Isotherm, Kinetics, and Thermodynamics Analyses ,Polymers , Vol. 12 (10), 2020, https://doi.org/https://doi.org/10.3390/polym12102353
, Synthesis, characterization and application of a bio-derived ZnO nanopowder from spinacia oleracea leaf extract for the removal of BOD and COD from tannery wastewater ,Chemistry of the Total Environment , Vol. 1 (1),pp 18 , 2021
, A Comparative study of electro and chemical coagulation for efficient removal of lignin and some other pollutants from industrial wastewater ,Egyptian Journal of Chemistry , Vol. 63 (10),pp 40834093 , 2020, https://doi.org/https://doi.org/10.21608/ejchem.2020.29652.2811
, Treatment of industrial wastewater with gamma irradiation for removal of organic load in terms of biological and chemical oxygen demand ,Heliyon , Vol. 7 (2),pp e05972 , 2021, https://doi.org/https://doi.org/10.1016/j.heliyon.2021.e05972
, Toxicity of Ochratoxin to Early Life Stages of Zebrafish (Danio rerio) ,Toxins , Vol. 10 (7), 2018, https://doi.org/https://doi.org/10.3390/toxins10070264
, Doseresponse regressions for algal growth and similar continuous endpoints: Calculation of effective concentrations ,Environmental Toxicology and Chemistry , Vol. 28 (4),pp 826835 , 2009, https://doi.org/https://doi.org/10.1897/08-068R.1
, Physiological and molecular responses of the earthworm (Eisenia fetida) to soil chlortetracycline contamination ,Environmental Pollution , Vol. 171 ,pp 4651 , 2012, https://doi.org/https://doi.org/10.1016/j.envpol.2012.07.020
, Binary and ternary toxicological interactions of clothianidin and eight commonly used pesticides on honey bees (Apis mellifera) ,Ecotoxicol Environ Saf , Vol. 223 ,pp 112563 , 2021, https://doi.org/https://doi.org/10.1016/j.ecoenv.2021.112563
, Effects of acute ammonia exposure on antioxidant and detoxification metabolism in clam Cyclina sinensis ,Ecotoxicology and Environmental Safety , Vol. 211 ,pp 111895 , 2021, https://doi.org/https://doi.org/10.1016/j.ecoenv.2021.111895
, Models for the acute and chronic aqueous toxicity of vanadium to Daphnia pulex under a range of surface water chemistry conditions ,Ecotoxicology and Environmental Safety , Vol. 179 ,pp 301309 , 2019, https://doi.org/https://doi.org/10.1016/j.ecoenv.2019.04.052
, The toxic effects of three active pharmaceutical ingredients (APIs) with different efficacy to Vibrio fischeri ,Emerging Contaminants , Vol. 5 ,pp 297302 , 2019, https://doi.org/https://doi.org/10.1016/j.emcon.2019.08.004
, Enhanced Removal and Toxicity Decline of Diclofenac by Combining UVA Treatment and Adsorption of Photoproducts to Polyvinylidene Difluoride ,Polymers , Vol. 12 (10), 2020, https://doi.org/https://doi.org/10.3390/polym12102340
, Ecological assessment of coal mine and metal mine drainage in South Korea using Daphnia magna bioassay ,SpringerPlus , Vol. 4 (1),pp 518 , 2015, https://doi.org/https://doi.org/10.1186/s40064-015-1311-1
, Evaluation of the treatment process of landfill leachate using the toxicity assessment method ,International Journal of Environmental Research and Public Health , Vol. 13 (12), 2016, https://doi.org/https://doi.org/10.3390/ijerph13121262
, Data on effluent toxicity and physicochemical parameters of municipal wastewater treatment plant using Daphnia Magna ,Data Brief , Vol. 19 ,pp 18371843 , 2018, https://doi.org/https://doi.org/10.1016/j.dib.2018.06.076
, Detoxification of glucose, ammonium and formaldehyde using nitrification and plant processes ,Nature Environment and Pollution Technology , Vol. 19 (1), 2020
, Valorization of a pharmaceutical organic sludge through different composting treatments ,Waste Management , Vol. 74 ,pp 203212 , 2018, https://doi.org/https://doi.org/10.1016/j.wasman.2017.12.017
, Respiration and enzymatic activities as indicators of stabilization of sewage sludge composting ,Waste Management , Vol. 39 ,pp 104110 , 2015, https://doi.org/https://doi.org/10.1016/j.wasman.2015.01.028
, Furfural Biodegradation in Consortium through Bacillus licheniformis, Microbacterium sp. and Brevundimonas sp. ,Journal of Sustainable Development of Energy, Water and Environment Systems , Vol. 10 (1),pp 110 , 2022, https://doi.org/https://doi.org/10.13044/j.sdewes.d9.0392
, Dimer formation during UV photolysis of diclofenac ,Chemosphere , Vol. 93 (9), 2013, https://doi.org/https://doi.org/10.1016/j.chemosphere.2013.06.079
, A photochemical study of diclofenac and its major transformation products ,Photochemistry and Photobiology , Vol. 86 (3), 2010, https://doi.org/https://doi.org/10.1111/j.1751-1097.2009.00703.x
, Towards sustainable and energy efficient municipal wastewater treatment by up-concentration of organics ,Progress in Energy and Combustion Science , Vol. 70 ,pp 145168 , 2019, https://doi.org/https://doi.org/10.1016/j.pecs.2018.10.002
, Continuous self-provided fermentation for microbial lipids production from acetate by using oleaginous yeasts Cryptococcus podzolicus and Trichosporon porosum ,Renewable Energy , Vol. 146 ,pp 737743 , 2020, https://doi.org/https://doi.org/10.1016/j.renene.2019.07.013
, Vibrio fischeri Bioassay for Determination of Toxicity in Petroleum Contaminated Soils from Tropical Southeast Mexico ,Sains Malaysiana , Vol. 44 (3), 2015, https://doi.org/https://doi.org/10.17576/jsm-2015-4403-04
, Comparison of joint effect of acute and chronic toxicity for combined assessment of heavy metals on Photobacterium sp.NAA-MIE ,International Journal of Environmental Research and Public Health , Vol. 18 (12), 2021, https://doi.org/https://doi.org/10.3390/ijerph18126644
, The mixture toxicity of heavy metals on Photobacterium phosphoreum and its modeling by ion characteristics-based QSAR ,PLoS ONE , Vol. 14 (12), 2019, https://doi.org/https://doi.org/10.1371/journal.pone.0226541
, Landfill leachate treatment by electrochemical oxidation ,Waste Management , Vol. 29 (9),pp 25342541 , 2009, https://doi.org/https://doi.org/10.1016/j.wasman.2009.05.004
, Electrocoagulation process to Chemical and Biological Oxygen Demand treatment from carwash grey water in Ahvaz megacity, Iran ,Data in Brief , Vol. 11 ,pp 634639 , 2017, https://doi.org/https://doi.org/10.1016/j.dib.2017.03.006
, Ozone pretreatment of biomethanated distillery wastewater in a semi batch reactor: mapping pretreatment efficiency in terms of COD, color, toxicity and biohydrogen generation ,Biofuels , Vol. 11 (7),pp 801809 , 2020, https://doi.org/https://doi.org/10.1080/17597269.2017.1416521
, Enhancing Biodegradability of Textile Wastewater by Ozonation Processes: Optimization with Response Surface Methodology ,Ozone: Science & Engineering , Vol. 40 (6),pp 465472 , 2018, https://doi.org/https://doi.org/10.1080/01919512.2018.1474339
, Effects of ozonation process on lignin-derived compounds in pulp and paper mill effluents ,Journal of Hazardous Materials , Vol. 142 (1),pp 250257 , 2007, https://doi.org/https://doi.org/10.1016/j.jhazmat.2006.08.011
, Efficiency of fenton process in olive oil mill wastewater treatment ,Journal of Mazandaran University of Medical Sciences , Vol. 29 (176), 2019
, Natural Gas Processing Wastewater Management ,Egyptian Journal of Chemistry , Vol. 62 (1),pp 6373 , 2019, https://doi.org/https://doi.org/10.21608/ejchem.2019.12230.1763
, Biodegradability enhancement of real antibiotic metronidazole wastewater by a modified electrochemical Fenton ,Journal of the Taiwan Institute of Chemical Engineers , Vol. 96 ,pp 256263 , 2019, https://doi.org/https://doi.org/10.1016/j.jtice.2018.11.019
, Application of electro-Fenton process for treatment of composting plant leachate: Kinetics, operational parameters and modeling ,Journal of Environmental Health Science and Engineering , Vol. 17 (1), 2019, https://doi.org/https://doi.org/10.1007/s40201-019-00361-2
, Application of Photo-Fenton Process for the Treatment of Kraft Pulp Mill Effluent ,Advances in Chemical Engineering and Science , Vol. 4 (4), 2014, https://doi.org/https://doi.org/10.4236/aces.2014.44050
, Obtaining Plant and Soil Biostimulants by Waste Whey Fermentation ,Waste Biomass Valor , Vol. 11 (7),pp 32813292 , 2020, https://doi.org/https://doi.org/10.1007/s12649-019-00660-7
, Growth, biostimulant and biopesticide activity of the MACC-1 Chlorella strain cultivated outdoors in inorganic medium and wastewater ,Algal Research , Vol. 53 ,pp 102136 , 2021, https://doi.org/https://doi.org/10.1016/j.algal.2020.102136
, Plant stimulants and horticultural production ,MOJ Ecology & Environmental Sciences , Vol. 5 (6),pp 261265 , 2020, https://doi.org/https://doi.org/10.15406/mojes.2020.05.00202
, Plasmid-Mediated Bioaugmentation for the Bioremediation of Contaminated Soils ,Frontiers in Microbiology , Vol. 8 ,pp 1966 , 2017
, Bioaugmentation and biostimulation: a potential strategy for environmental remediation ,Journal of Microbiology & Experimentation , Vol. 6 (5),pp 223231 , 2018, https://doi.org/https://doi.org/10.15406/jmen.2018.06.00219
, Effect of bioaugmentation of activated sludge with white-rot fungi on olive mill wastewater detoxification ,Letters in Applied Microbiology , Vol. 42 (4), 2006, https://doi.org/https://doi.org/10.1111/j.1472-765X.2006.01858.x
, Biodegradation by bioaugmentation of dairy wastewater by fungal consortium on a bioreactor lab-scale and on a pilot-scale ,Journal of Environmental Sciences , Vol. 25 (9),pp 19061912 , 2013, https://doi.org/https://doi.org/10.1016/S1001-0742(12)60239-3
, Enhancement of biological treatment of industrial wastewater using bioaugmentation technology ,Technologies and Management for Sustainable Biosystems ,pp 2534 , 2010
, The cocoa bean fermentation process: from ecosystem analysis to starter culture development ,Journal of Applied Microbiology , Vol. 121 (1),pp 517 , 2016, https://doi.org/https://doi.org/10.1111/jam.13045
, Bioaugmented Mixed Culture by Clostridium aceticum to Manipulate Volatile Fatty Acids Composition From the Fermentation of Cheese Production Wastewater ,Frontiers in Microbiology , Vol. 12 ,pp 658494 , 2021, https://doi.org/https://doi.org/10.3389/fmicb.2021.658494
, Promising approach for composting disposable diapers enhanced by Cyanobacteria ,Global Journal of Environmental Science and Management , Vol. 7 (3),pp 439456 , 2021, https://doi.org/https://doi.org/10.22034/GJESM.2021.03.08
, Phytoremediation of emulsion paint wastewater using Azolla Pinnata, Eichhornia Crassipes and Lemna Minor ,Nigerian Journal of Technology , Vol. 40 (3), 2021, https://doi.org/https://doi.org/10.4314/njt.v40i3.21
, The Efficiency of Lemna minor L. in the Phytoremediation of Romi Stream: A Case Study of Kaduna Refinery and Petrochemical Company Polluted Stream ,J App Biol Biotech , Vol. 3 (1),pp 1114 , 2015, https://doi.org/https://doi.org/10.7324/JABB.2015.3102
, Phragmites australis a helophytic grass can establish successful partnership with phenol-degrading bacteria in a floating treatment wetland ,Saudi Journal of Biological Sciences , Vol. 26 (6),pp 11791186 , 2019, https://doi.org/https://doi.org/10.1016/j.sjbs.2018.01.014
, Assessment of extended aeration sludge process in Jahra wastewater treatment plant-Kuwait ,International Journal of Environmental Science and Development , Vol. 10 (2), 2019, https://doi.org/https://doi.org/10.18178/ijesd.2019.10.2.1148
, Treatment and potential reuse of greywater from schools: a pilot study ,Water Science and Technology , Vol. 75 (9),pp 21192129 , 2017, https://doi.org/https://doi.org/10.2166/wst.2017.088
, A combined activated sludge-filtration-ozonation process for abattoir wastewater treatment ,Journal of Water Process Engineering , Vol. 25 ,pp 157163 , 2018, https://doi.org/https://doi.org/10.1016/j.jwpe.2018.07.009
, Treatment of municipal landfill leachate at different stabilization stages in two-stage membrane bioreactor bioaugmented with Alcaligenes faecalis no. 4 ,Bioresource Technology Reports , Vol. 11 ,pp 100528 , 2020, https://doi.org/https://doi.org/10.1016/j.biteb.2020.100528
, Phytoremediation potential of Vetiver system technology for improving the quality of palm oil mill effluent ,Advances in Materials Science and Engineering , Vol. 2014 ,pp 683579 , 2014, https://doi.org/https://doi.org/10.1155/2014/683579
, Characterization and Treatment of Textile Wastewater by Aquatic Plants (Macrophytes) and Algae ,EUR J SUSTAIN DEV RES , Vol. 2 (3),pp 29 , 2018, https://doi.org/https://doi.org/10.20897/ejosdr/85933
, Development and evaluation of a constructed pilot-scale horizontal subsurface flow wetland treating piggery wastewater ,Ain Shams Engineering Journal , Vol. 9 (4),pp 31793185 , 2018, https://doi.org/https://doi.org/10.1016/j.asej.2018.04.002
, Assessment of autochthonous aquatic macrophytes with phytoremediation potential for dairy wastewater treatment in floating constructed wetlands ,International Journal of Phytoremediation , Vol. 22 (5),pp 518528 , 2020, https://doi.org/https://doi.org/10.1080/15226514.2019.1686603
, Phytoremediation as a tool for the remediation of wastewater resulting from dyeing activities ,Applied Ecology and Environmental Research , Vol. 17 (2), 2019, https://doi.org/https://doi.org/10.15666/aeer/1702_37233735
, Use of native aquatic macrophytes in the reduction of organic matter from dairy effluents ,International Journal of Phytoremediation , Vol. 19 (8),pp 781788 , 2017, https://doi.org/https://doi.org/10.1080/15226514.2017.1284750
, Integrated Design of a Small Wastewater Treatment Plant - A Case Study from Thailand ,Journal of Sustainable Development of Energy, Water and Environment Systems , Vol. 10 (2),pp 119 , 2022, https://doi.org/https://doi.org/10.13044/j.sdewes.d8.0379
, Suitability of Macrophytes for Wastewater Treatment and Biogas Generation ,International Journal of Innovative Technology and Exploring Engineering , Vol. 9 (2S2), 2019, https://doi.org/https://doi.org/10.35940/ijitee.b1005.1292s219
, Design of a Reed Bed System for Treatment of Domestic Wastewater using Native Plants ,J. Ecol. Eng. , Vol. 21 (6),pp 2228 , 2020, https://doi.org/https://doi.org/10.12911/22998993/123256
, Phytoremediation of industrial mines wastewater using water hyacinth ,International Journal of Phytoremediation , Vol. 19 (1),pp 8796 , 2017, https://doi.org/https://doi.org/10.1080/15226514.2016.1216078
, Urgent need of wastewater treatment based on BOD footprint for aerobic conditions of receiving water ,Journal of Applied Sciences Research , Vol. 8 (1),pp 454457 , 2012
, The Effectiveness of Microbubble Technology in The Quality Improvement of Raw Water Sample ,IOP Conf. Ser.: Mater. Sci. Eng. , Vol. 1144 (1),pp 012053 , 2021, https://doi.org/https://doi.org/10.1088/1757-899X/1144/1/012053
, Municipal wastewater treatment in horizontal and vertical flows constructed wetlands ,Ecological Engineering , Vol. 61 ,pp 460468 , 2013, https://doi.org/https://doi.org/10.1016/j.ecoleng.2013.10.010
, Biological treatment of dairy wastewater using activated sludge ,ScienceAsia , Vol. 39 (2),pp 179 , 2013, https://doi.org/https://doi.org/10.2306/scienceasia1513-1874.2013.39.179
, Water Quality Performance of a Batch-Type Stormwater Detention Basin ,Water Environment Research , Vol. 80 (2),pp 172178 , 2008, https://doi.org/https://doi.org/10.2175/106143007X220842
, Potential of Duckweed for Swine Wastewater Nutrient removal and Biomass Valorisation through Anaerobic Co-digestion ,Journal of Sustainable Development of Energy, Water and Environment Systems. , Vol. 5 (2),pp 127138 , 2017, https://doi.org/https://doi.org/10.13044/j.sdewes.d5.0137
, Evaluation of an Organic Waste Composting Device to Household Treatment ,Journal of Sustainable Development of Energy, Water and Environment Systems. , Vol. 3 (3),pp 245255 , 2015, https://doi.org/https://doi.org/10.13044/j.sdewes.2015.03.0019
, Anaerobic baffled reactor to treat fishmeal wastewater with high organic content ,Environmental Technology & Innovation , Vol. 17 ,pp 100586 , 2020, https://doi.org/https://doi.org/10.1016/j.eti.2019.100586
, Field-scale anaerobic baffled reactor for domestic wastewater treatment: effect of dynamic operating conditions ,Water Practice and Technology , Vol. 16 (1),pp 4258 , 2020, https://doi.org/https://doi.org/10.2166/wpt.2020.103
, Swine wastewater treatment using an anaerobic baffled (ARB) and a UASB reactor system ,Semina: Ciências Agrárias , Vol. 38 (6), 2017, https://doi.org/https://doi.org/10.5433/1679-0359.2017v38n6p3705
, Anaerobic Baffled Reactor (ABR) for Wastewater Treatment ,Environmental Anaerobic Technology ,pp 163184 , 2010, https://doi.org/https://doi.org/10.1142/9781848165434_0008
, Optical and Structural Characterization of Bi2FexNbO7 Nanoparticles for Environmental Applications ,Emerging Science Journal , Vol. 4 (1), 2020, https://doi.org/https://doi.org/10.28991/esj-2020-01205
, Series Type Vertical Subsurface Flow Constructed Wetlands for Dairy Farm Wastewater Treatment ,Civil Engineering Journal , Vol. 7 (2), 2021, https://doi.org/https://doi.org/10.28991/cej-2021-03091654
, Current issues confounding the rapid toxicological assessment of oil spills ,Chemosphere , Vol. 245 ,pp 125585 , 2020, https://doi.org/https://doi.org/10.1016/j.chemosphere.2019.125585
, Bioassay evaluation of toxicity reduction in common effluent treatment plant ,Journal of Applied and Natural Science , Vol. 1 (1), 2009, https://doi.org/https://doi.org/10.31018/jans.v1i1.23
, Monitoring of the ecotoxicological hazard potential by polar organic micropollutants in sewage treatment plants and surface waters using a mode-of-action based test battery ,J. Environ. Monit. , Vol. 10 (5),pp 622631 , 2008, https://doi.org/https://doi.org/10.1039/B800951A
, Monitoring biological wastewater treatment processes: recent advances in spectroscopy applications ,Rev Environ Sci Biotechnol , Vol. 16 (3),pp 395424 , 2017, https://doi.org/https://doi.org/10.1007/s11157-017-9439-9
, Monitoring Aquaculture Water Quality: Design of an Early Warning Sensor with Aliivibrio fischeri and Predictive Models ,Sensors , Vol. 18 (9), 2018, https://doi.org/https://doi.org/10.3390/s18092848
, Development and implementation of microbial sensors for efficient process control in wastewater treatment plants ,Bioprocess Biosyst Eng , Vol. 31 (3),pp 277282 , 2008, https://doi.org/https://doi.org/10.1007/s00449-007-0192-2
, Sorting the main bottlenecks to use paper-based microbial fuel cells as convenient and practical analytical devices for environmental toxicity testing ,Chemosphere , Vol. 265 ,pp 129101 , 2021, https://doi.org/https://doi.org/10.1016/j.chemosphere.2020.129101
, Monitoring a municipal wastewater treatment process using a trend analysis ,Environmental Technology , Vol. 39 (24),pp 31933202 , 2018, https://doi.org/https://doi.org/10.1080/09593330.2017.1375026
, Research strategy on kenaf for phytoremediation of organic matter and metals polluted soil. ,Advances in Environmental Biology , Vol. 8 (17),pp 6467 , 2014
, Phytoremediation advances in the lab but lags in the field ,Proceedings of the National Academy of Sciences , Vol. 114 (29),pp 74757477 , 2017, https://doi.org/https://doi.org/10.1073/pnas.1707883114
, Mixed plant operations for phytoremediation in polluted environments a critical review ,Journal of Phytology , Vol. 12 ,pp 99103 , 2020, https://doi.org/https://doi.org/10.25081/jp.2020.v12.6454
, Bioremediation of PAH-Contaminated Soils: Process Enhancement through Composting/Compost ,Applied Sciences , Vol. 10 (11), 2020, https://doi.org/https://doi.org/10.3390/app10113684
, Landscape Intervention Design Strategy with Application of Islamic Ornamentation at Trunojoyo Park Malang, Jawa Timur, Indonesia ,Journal of Islamic Architecture , Vol. 6 (1), 2020, https://doi.org/https://doi.org/10.18860/jia.v6i1.4383
, Treatment Technology of Microbial Landscape Aquatic Plants for Water Pollution ,Advances in Materials Science and Engineering , Vol. 2021 ,pp e4409913 , 2021, https://doi.org/https://doi.org/10.1155/2021/4409913
, Screening of Indigenous Microorganisms as Potential Biofertilisers for Periurban Horticulture Areas ,Journal of Sustainable Development of Energy, Water and Environment Systems , Vol. 10 (1),pp 1.-14. , 2022, https://doi.org/https://doi.org/10.13044/j.sdewes.d8.0350
, Furfural Biodegradation in Consortium through Bacillus licheniformis, Microbacterium sp. and Brevundimonas sp. ,Journal of Sustainable Development of Energy, Water and Environment Systems , Vol. 10 (1),pp 1.-10. , 2022, https://doi.org/https://doi.org/10.13044/j.sdewes.d9.0392
, Rhizosphere processes and management in plant-assisted bioremediation (phytoremediation) of soils ,Plant Soil , Vol. 321 (1),pp 385408 , 2009, https://doi.org/https://doi.org/10.1007/s11104-008-9686-1
, Phytoextraction, phytotransformation and rhizodegradation of ibuprofen associated with Typha angustifolia in a horizontal subsurface flow constructed wetland ,Water Research , Vol. 102 ,pp 294304 , 2016, https://doi.org/https://doi.org/10.1016/j.watres.2016.06.049
, Soil conditioners improve rhizodegradation of aged petroleum hydrocarbons and enhance the growth of Lolium multiflorum ,Environ Sci Pollut Res , Vol. 29 (6),pp 90979109 , 2022, https://doi.org/https://doi.org/10.1007/s11356-021-16149-7
,